Research project Personalised treatment planning for stereotactic radiotherapy of high-grade glioma
Stereotactic radiotherapy is a highly successful radiotherapy modality for specific types of cancer involving the delivery of the treatment in one (radiosurgery) or very few sessions.
Stereotactic radiotherapy is a highly successful radiotherapy modality for specific types of cancer involving the delivery of the treatment in one (radiosurgery) or very few sessions. It relies on the accurate definition of the volumes confining the malignant cells and the organs at risk of being irradiated as a very high radiation dose is planned to be delivered to the target in order to ensure the sterilisation of the malignant clonogenic cells. There are, however, multiple instances when the treatment fails to be successful or it is denied to patients because of the uncertainties in target position and extent. One of the most problematic brain tumours in that respect is glioma, in particular high-grade glioma. Glioblastoma, for instance, are very well known for infiltrating the brain tissue far from the observable tumour mass on the diagnostic images. A new philosophy for defining the target for glioma and a corresponding new approach for clinically handling it are now emerging in the form of personalised probabilistic target definition and treatment planning aiming at ensuring a robust delivery of the dose with respect to the target definition and hence patient cure.
It is therefore the overall aim of this project to develop the framework for personalised treatment planning for stereotactic radiotherapy accounting for uncertainties in target definition and position for high-grade glioma.
The specific aims of the project are:
1. To develop the methods and the computational tools for personalised probabilistic planning accounting for uncertainties in target definition, extent and position for high-grade glioma.
2. To validate the model for probabilistic target definition on a cohort of gliobastoma patients and to compare the results with the current state-of-the-art definition of the target.
3. To test the model for target definition on a subset of cases of glioblastoma.
It is expected that based on better target definition and individualised probabilistic and robust treatment plans, this project will contribute to the increase of the success of radiotherapy and therefore an increase of the overall survival of glioblastoma patients which is currently less than 15 months on average.
Project description
Delivering a highly conformal dose distribution to the target in one fraction allowing at the same time the sparing of the normal tissue and the critical structures is part of the basic concept of stereotactic radiosurgery (SRS). Provided that the highly accurate radiosurgical equipment available today is used, such as the Gamma Knife or the advanced linac-based technologies, planning and delivering the prescribed dose distribution is an achievable goal, and therefore the main issue that remains to be solved is the definition of the target. As the planning target volume in radiosurgery was historically defined without margins, because of the accurate stereotactic definition of the coordinate system and rigid patient immobilisation, the success of the stereotactic approach critically depends on accurate target delineation. This could be identified as a factor of key importance in radiosurgery. The current view in SRS regarding the target volume is binary in the sense that it is assumed that the target cells are confined with certainty within the delineated border and hence the treatment planning optimisation aims at a high coverage of the target volume with a prescribed dose and high selectivity hence a steep falloff of the dose distribution outside of the target volume. This appears to neglect, however, the microscopic tumour spread and does not reflect the uncertainties in the extent, shape and position of the tumour. Extensive work was therefore performed in our group on assessing the variability in contouring the targets and hence defining the targets in radiosurgery. More specifically, the multi-observer variability of delineation for radiosurgery targets was analysed and quantified. The potential impact of the differences in target delineation with respect to tumour control probability was also investigated and a robust method for estimating the true target for individual cases based on multiple delineations was developed. The possibility of accounting for the errors in target delineation through probabilistic and robust treatment planning has therefore started to be investigated. The previous work on the assessment of the variability in target definition revealed the fact that some radiosurgery targets, such as high-grade gliomas, are more prone than others to present high variability in the delineation of the volume to be irradiated. Glioblastomas, tumours with a well-documented infiltrative character into the normal tissues, are therefore a typical example for these variability-prone targets. Indeed, defining the target for glioblastoma is not a trivial task but an individual critical clinical problem as tumour cells infiltrate the brain tissue beyond the abnormality visible on magnetic resonance imaging (MRI) scans. Isolated tumour cells could be found by biopsy tests outside of hyperintensity volume on T2-weighted MRI scans and consequently 80% of the recurrences are within a 2 cm margin of the contrast enhanced lesion. Consequently, the European Organisation for Research and Treatment of Cancer (EORTC) guidelines for the delineation of high-grade gliomas recommend for a single target a dose biologically equivalent to 60 Gy in 2 Gy per fraction to be delivered to a clinical target volume defined as a 2 cm isotropic expansion of the volume delineated based on a contrast enhancing T1-weighted MRI scan. The 2 cm margin is also recommended by the Radiation Therapy Oncology Group (RTOG) in USA. This large margin limits the choices regarding the radiotherapy modalities to be used in the treatment of glioblastoma in favour of linac-based fractionated radiotherapy with moderate success. Indeed, the average number of astrocytoma/glioblastoma cases treated with the radiosurgery-dedicated Gamma Knife technique at Karolinska University Hospital, for example, has decreased from 5.0±1.7 % of the total number of diagnoses between 2008 and 2018 to only 1.8% in 2019, one of the main reasons for this drop being the uncertainty in the definition of the target extent because of the infiltration into the normal tissue. The current clinical experience indeed suggests that improvements in radiotherapy alone will not lead to patient cure. However, in terms of effectiveness, radiotherapy against glioblastoma is the leading form of therapy contributing to prolonging the patient survival. Improved target definition might therefore have the potential to increase the capability of radiotherapy to manage glioma and possibly increase patient survival and reduce the treatment related toxicity. While understanding microscopic tumour progression generally requires an interdisciplinary effort involving radiation oncologists, radiologists, pathologists and surgeons, there is a role for medical physicists and computer scientists in the definition of the clinical target beyond imaging through the use of computational methods. This fact was recognised within the European SocieTy for Radiotherapy and Oncology (ESTRO) and a work group on Target definition transformed from an art to a science has been established. The mission of this work group is to replace the cumbersome manual process of defining the Clinical Target Volume (CTV) with a largely computerized process that allows user adjustments and to define steps/data/questions to create a personalized approach to define the CTV. The core group of leading scientists on this initiative consists of Prof Jan Unkelbach (Group leader Medical Physics Research, University of Zurich, Switzerland), Prof Vincent Grégoire (Head of the Radiation Oncology Department at the Léon Bérard Cancer Center, Lyon, France), Dr Cristina Garibaldi (Department of Medical Physics, European Institute of Oncology, Milan, Italy), Dr Nick Reynaert (Medical Physics Department, Institut Jules Bordet, Brussels, Belgium), Prof Thomas Bortfeld (Chief of Physics Division, Massachusetts General Hospital, Boston MA, US), Prof Marcel van Herk (Chair in Radiotherapy Physics, University of Manchester, UK) and Prof Iuliana Toma-Dasu (Department of Physics, Stockholm University). Considerable clinical effort has been made to image glioblastoma in the attempt to better define the radiotherapy target. Functional imaging modalities such as Positron Emission Tomography (PET) using Fluoro-Ethyl-Tyrosine(FET) and Methionine (MET) used in conjunction with MRI are showing promises for improving the definition of the GTV. They, however, are not able to map the areas of tumour cell infiltration far from the observable tumour border visible on MRI. Consequently, several mathematical models aiming at reproducing glioma’s complex spatial growth patterns have been developed but their clinical impact has so far been very limited. Along this line, a model for high-grade gliomas with a widespread infiltration beyond the lesion detectable on diagnostic images was developed within our group. Treatment plans for Gamma Knife radiosurgery (GKRS) with different combinations of dose prescription and target extents were designed and evaluated using standard conformity indexes (CI) and radiobiological parameters. Considerable differences in terms of tumour control probability were found between plans having similar CI but different targets. In conclusion, to account for tumour cell infiltration outside the target is of key importance in GKRS and a radiobiological evaluation should accompany well-established CI. Depending on the size of the target, radiosurgery delivered in one singe fraction might not always be the treatment of choice despite of its undoubtable radiobiological advantages because of the constraints to be imposed by the organs at risk (OARs). The development of the new generation of the GK device with a frameless immobilization system has not only increased the comfort of the patient by removing the stereotactic frame but has also opened the possibility to perform fractionated treatments adding therefore up to the treatment techniques options for delivering fractionated radiotherapy to glioma patients. This new opportunity, however, brings in addition to the incontestable advantages a reason of concern regarding the potential patient movement within the mask frame, as for linac-based radiosurgery, and hence target displacement relative to the planning position. Limited information is available regarding the extent of movement and therefore a recent study was initiated by the main applicant and her collaborators together with the GK unit at the Radiation Oncology department, Princess Alexandra Hospital, Brisbane, Australia, aiming at finding the uncertainties in target localization. These errors and uncertainties in the definition and the position of the target might adversely affect the treatment outcome and therefore need to be addressed whether the treatment is to be performed with the GK or with the linac-based equipment. The present research proposal aims towards taking advantage of the knowledge regarding these uncertainties by using them as input for personalised probabilistic planning aiming at delivering robust dose distributions. The overall aim of this project is therefore to increase the probability of controlling the tumour for high-grade gliomas by taking into account through probabilistic planning several key factors in stereotactic radiotherapy: the uncertainties in target extent for tumours of infiltrative character and of the uncertainties in target position during the delivery of the treatment. |
The idea of creating an in silico testbed for radiotherapy based on a virtual tumour model that would account for the main tumour microenvironmental features known to be related to the outcome of radiotherapy integrated into a treatment planning and optimisation system has a very high novelty factor, as, to the best of knowledge of the applicant, it has never been presented. The in silico investigation is extremely important as it could help designing clinical trials aiming at optimising the patient selection for different forms of radiotherapy and hence maximising the benefit for the patients by in silico assessing the relative clinical effectiveness of proton or ion radiotherapy compared to the common practice involving photon therapy, The theoretical modelling of an in silico tumour has also the potential to identify fast the relative importance of the factors that determine the response to treatment and hence help designing the clinical trials, before or even without lengthy and costly experimental studies in animal models. Currently, many pre-clinical studies are carried out on animals on which tumours are induced and allowed to develop. An ethical question regarding the use of animals in (chemo)radiotherapy research therefore arises and consequently alternative solutions to animal experiments should always be sought. This Replacement principle of modern research has been specified together with Reduction and Refinement in the Directive 2010/63/EU on the protection of animals used for scientific purposes. In the era of virtual reality, tumour models created in silico have the potential to allow the progress in research minimising at the same time the use of animals for experiments, thus fulfilling the recommendations of Directive 2010/63/EU. |
Project members
Project managers
Iuliana Livia Dasu
Professor
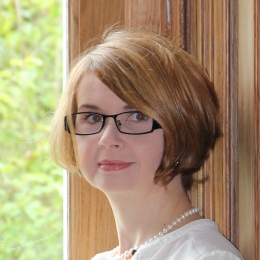
Members
Marta Lazzeroni
Universitetslektor
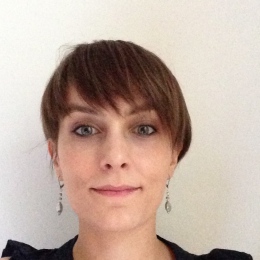